Is there such a thing as the intelligence gene? That of language? What about the one for creativity? As strange as these kinds of questions may seem, there was a time – a few decades ago now, thankfully – when the idea that our genes would determine what we are was present, sometimes among experts and much more often in popularized discourse. Genetics, in any case, is a highly developed and powerful branch of current biology. In addition to being fundamental to the understanding of life in all its manifestations, genetics has always been considered an important resource in medical research as well: it is enough to mention that Renato Dulbecco, as early as 1986, saw the project of sequencing the human genome as a "turning point" for cancer research. Current debates are full of talk about genes, from GMOs (genetically modified organisms) to gene therapies, up to ... viruses, the focus of attention in recent years ...
Now, the origin of all these developments must be found in the Augustinian Abbey of St. Thomas in Brünn (now Brno in the Czech Republic), where the naturalist friar Gregor J. Mendel (Hynčice, July 20, 1822 - Brno, January 6, 1884) carried out his experiments on the hybridization of pea plants (Pisum sativum). He hybridized thousands of plants for eight consecutive years, then carefully observed the passage of certain characteristics of the seeds of these plants from generation to generation. These are the famous yellow and wrinkled or green and smooth peas that we have probably all heard about at school. The results of Mendel's studies were then made public on February 8, 1865, in a lecture entitled "Experiments in Plant Hybridization", that he delivered at the Brünn Natural History Society, which Mendel himself had helped found four years earlier.
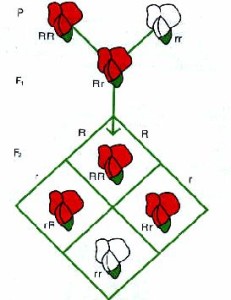
At the top the pure lines, dominant and recessive, and the dominant hybrids obtained from their crossing. At the bottom, the distribution of phenotypes and genotypes of the third generation.
With his patient experimental work, Mendel succeeded in producing so-called "pure lines" – that is, lines of plants in which certain characters remained constant for many generations. Thus the naturalist friar had at his disposal, for example, pure lines of yellow-seeded pea plants and pure lines of green-seeded pea plants. Therefore, Mendel crossed yellow-seeded plants with green-seeded plants. The plants generated from this cross all showed yellow seeds. This allowed Mendel to formulate his first law, the law of dominance and uniformity. According to this law, crossings obtained from pure lines for a character will show only the dominant character. In our example, the yellow character of the seed will be the dominant one, while the green character, which does not show itself in the first generation of crossings, will be the recessive one. These terms were introduced by Mendel himself. At this point, continuing his patient research work, Mendel cross-bred the plants obtained by crossing the two pure lines with each other and noticed that, in this next generation the recessive character, which had disappeared in the first generation obtained by crossing the pure lines, reappeared. More exactly, Mendel noted that the recessive character reappeared with a precise proportion: "3:1" – that is, of all the plants obtained by crossing plants that were themselves obtained by crossing pure lines, one quarter returned to show the recessive character and three quarters continued to show the dominant character. Other results, which it would be complicated to detail here, would also suggest to Mendel that in this third generation, beyond the characters exhibited by the plants according to the 3:1 proportion, the determinants ("anlage," in Mendel's original terminology) were distributed according to this proportion: "1YY:2Yg:1gg" – that is, one quarter of the plants had both determinants for the dominant character (Y, yellow), half had one dominant determinant (Y) and one recessive determinant (g, green) and thus showed the dominant character but also contained the recessive character, and one quarter had both recessive determinants (g) and thus showed the recessive character. Mendel arrived at this result by analyzing still successive generations of crosses and studying the proportions with which the characters showed (phenotypically). This result led Mendel to his second law, the law of segregation, according to which the determinants present in the parental generation separate discretely in producing gametes, and then recombine precisely according to the 1:2:1 proportion. It is also worth mentioning Mendel's third law, the law of independent assortment: during gamete formation, the determinants for a certain character (e.g., yellow or green color) are distributed independently of the determinants for another character (e.g., smooth or wrinkled seed surface).
Now, these results led Mendel to believe that the determinants were material elements present in the gametes of plants and that they were transmitted as discrete material units: "This development follows a constant law, based on the material composition and distribution of the elements encountered in the cell in a vital union" – as we read in the concluding remarks of the account read by Mendel in February 1865 before the Natural History Society of Brünn. After all, this is the root of the distinction between phenotype (the characters exhibited by the organism) and genotype (the characters encoded in the genes, whether they are dominant alleles – which therefore manifest phenotypically – or recessive alleles – which therefore can be carried by the gametes even when they do not manifest).
These elements – the "determinants" – would later become, in the early 1900s, the "genes" – and, essentially, this the very reason why Mendel is today considered the father of genetics. His, in fact, was the insight that the "determinants" of traits were transmitted from generation to generation through discrete material particles, which, as such, could be transmitted across generations even when their effects (the traits associated with them) did not exhibit themselves in a particular generation. Mendel's work – perhaps because of its novelty or of the mathematical format it was given – had no particular consequences in the scientific world for more than three decades. It was not until the year 1900 that the Mendelian account of 1865 was given primacy in the formulation of the laws of genetics by the Tübingen botanist Carl Correns in the context of a discussion with another prominent Dutch botanist, Hugo de Vries. In 1901 Mendel's account was translated into English by William Bateson, a distinguished British geneticist who six years later, in 1906, first used the term "genetics", specifically in a lecture on plant hybridization.
Mendel's research is striking both for the rigor of his experimental investigations and for the application of mathematical methods to the analysis of the results obtained over the years on thousands and thousands of specimens. In short, an application in the biological field of the "manifest experiences and necessary demonstrations" of Galilean memory.
He is perhaps even more striking for the insightfulness in the conclusions he reaches. A great philosopher just after Mendel, Charles S. Peirce (1839-1914) emphasized abduction as a process at once inferential and intuitive by which explanatory hypotheses are formed; only through abduction human ingenuity is able to introduce some new idea. According to Peirce, moreover, it is thanks to abduction that the existence of a fact completely different from anything observed can be inferred. In essence, this is perhaps the most important creative act a scientist can put forth: the formulation of theoretical entities. Well, Mendel did exactly that: he concluded the existence of his "determinants" as an explanation for the manifest behavior of pea plant traits over generations without being able to have any direct evidence of such material elements present in the gametes. Only with mid-20th-century developments in molecular biology evidence was found of the actual existence of these material elements, later "crystallized" in the notion of a "gene" as the coding portion of a DNA strand within a chromosome.
Another consideration should be stated explicitly. What was the research question that animated Mendel's patient studies? In essence, this question was evolutionary in nature – or at least, he took into account the challenge that the 19th-century developments in biology had already offered, first with the work of Lamarck and then with that of Darwin. The interest that had prompted Mendel to undertake his experimental research program was whether species are stable or transform over time. His results showed how biological variability was not unregulated and capricious but was accompanied by a substantial tendency to species stability – a central element then captured, in later developments, by the notion of "heredity." This question was probably aroused in him by his professors at the University of Vienna (where he studied from 1851), some of whom had already embraced an evolutionary conception of life, while others opposed it – this tension was certainly a great stimulus to the young Mendel.
The reader, at this point, might wonder about the relationship between Mendel and Darwin, who published On the Origin of Species shortly after Mendel began his experiments at the Brünn Monastery. There was, to the best of our knowledge, no direct contact or exchange between the two. Probably, Darwin never had the opportunity to learn about Mendel's work. Mendel, on the contrary, had the German versions of two of Darwin's works (On the Origin of Species and The Variation of Animals and Plants under Domestication), had read them and made notes on them. The issue of Darwinian influences on Mendel's works and of Mendel's views about Darwinian evolutionary theory are beyond the scope of the present context. Arguably, Mendel's reading of Darwin's work was subsequent to the beginning of his pea experiments, but almost certainly it was prior to 1865 – so that one could assume (in fact, one could almost prove it, according to D.J. Fairbanks' work "Mendel and Darwin: untangling a persistent dilemma", Heredity 124/2020: 263-273) that even if those readings did not motivate Mendel's research, they probably influenced the interpretation of the results.
What is certain is that the intertwinement of Darwin's theory of natural selection and Mendel's studies at the origin of genetics are the two pillars of that impressive theoretical construction elaborated in the central decades of the 20th century, known as the "neo-Darwinian synthesis," which has opened up immense scenarios in our understanding of the phenomenon of life and its evolution.
To think that the roots of one of the most important and consequence-rich developments on all levels (scientific, technological, medical, anthropological, ethical and interdisciplinary) lie in an Augustinian monastery is not without its fascination. Yet this is not just a curious coincidence. Mendel's entry into the Brünn monastery is an essential part of his research work. First, religious life gave Mendel the practical opportunity to "cultivate" his naturalistic interests – as well as thousands of pea plants. Second, the Brünn monastery – and particularly the abbot who welcomed Mendel to the monastery, Cyrill Napp (1792-1867) – encouraged scientific research and regarded study as the highest form of prayer. It even seems that the Brünn monastery was investigated for "secularism" at the time of Napp and Mendel precisely because of the emphasis put on the study of nature and the sciences. Be that as it may, the debt to Mendel is certainly to his genius, insight, and perseverance, but also to his being an Augustinian friar of the Brünn monastery.
Ivan Colagè,
Vice-Director of the DISF-Center
June 2022